- Balancing energy supply and demand in a system dominated by intermittent renewable generation energy sources is more challenging than one dominated by more dispatchable fossil generation.
- As more non-dispatchable – or intermittent – generation resources enter the grid, having dispatchable demand becomes more important.
- Efficiently supplying future baseload power requires a diversified grid that integrates advanced technologies for balancing supply and demand. This enhanced infrastructure should increase flexibility, facilitate higher penetrations of renewable generation, and improve system reliability.
A decade ago, the California Independent System Operator (CAISO), the entity responsible for overseeing about 80% of California’s electricity supply, coined the term “duck curve.” This was the first meaningful acknowledgment that balancing supply and demand in a system dominated by intermittent renewable generation energy sources would be significantly more challenging than one dominated by more dispatchable fossil generation. But over the last 10 years, the technologies available to utilities have proliferated. Now, renewables coupled with existing technologies such as demand response can provide baseload, intermediate load and peak load energy.
This article explores some of the technologies enabling this shift in power generation, especially those replacing traditional baseload resources such as coal and large-scale nuclear with newer more cost-effective, safer and cleaner technologies.
Flexibility and demand response will enable the energy transition
The energy transition will be dominated by a shift to increased electrification of buildings, transportation and commercial/industrial production. In the face of climate change, government policy and market forces are accelerating the energy transition. The US set a goal of having 50% of new vehicle sales be electric vehicles (EVs) by 2030 with other state and local municipalities setting more ambitious goals. Further electrification of buildings will occur as many cities, such as New York and San Francisco, have banned natural gas hookups in new construction. New York has gone a step further requiring energy retrofits on older buildings.
But what does increased electrification have to do with meeting baseload demand? In a word, flexibility. As increased penetrations of non-dispatchable (i.e. intermittent) generation resources enter the grid, having dispatchable demand becomes more important.
Enter demand response.
Using grid edge technologies, utilities can offer incentives or programs that enable customers to preheat water using electric water heaters or cool their homes, fridges and freezers while demand is low and supply is high. In effect, customers will use appliances as thermal batteries, so that later in the day when demand is high, those energy-intensive appliances are not running. The same is true for EVs but with the added benefit of being able to discharge power back to the grid through vehicle-to-grid (V2G) technology. Demand response technologies exist today for utilities, but further advances in demand response will continue.
Impulse Labs, for example, offers cooking appliances that run on lithium batteries to increase resilience for the consumer, decrease a home’s use of fossil fuel, and have the potential to enable demand response programs. Meanwhile other companies like Stem (commercial and industrial) and Swell (residential) are developing enhanced business models and software for deploying distributed assets. Another area primed for advancement is green hydrogen which could take advantage of low-cost electricity during periods of high renewable energy supply. This could be super charged by the significant incentives for green hydrogen in the Inflation Reduction Act of 2022 (IRA). Green hydrogen may also further the energy transition as it is used for high-temperature industrial process heat, transportation or power generation.
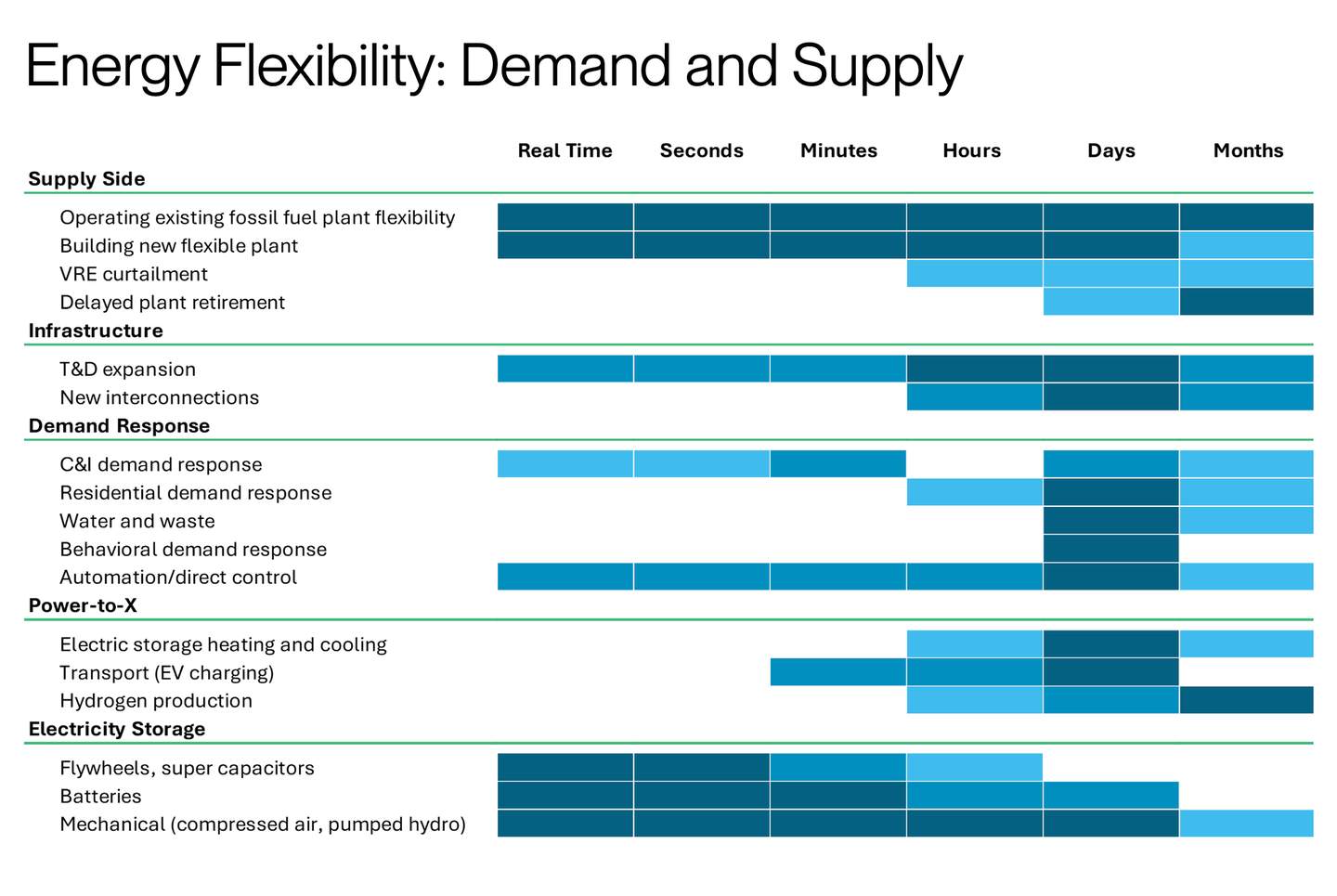
Enhanced grid edge technologies will bring demand response to life by improving utilities’ ability to monitor and control various distributed energy resources (DERs) through low latency sensor technology and edge computing. These advances will enable large-scale demand response and virtual power plants thus increasing penetrations of renewables on the grid. This will allow traditional renewable resources to replace baseload power – in addition to replacing expensive gas peaker plants used to meet short-term demand spikes. All this while increasing the reliability of the system.
Energy storage
Storage, both long-term and short-term term, must be a part of the energy transition. Storage technologies enhance grid flexibility and serve an important role in improving reliability by mitigating the intermittency of renewables. It also captures power during times of excess output and discharging that power during times of low supply.
Wind and solar are expected to account for over one third of all power generation by 2030, according to the Rocky Mountain Institute (RMI). With such a high percentage of energy coming from intermittent generation, scaling renewable energy will require safe, long-lasting and low-cost storage solutions. Bloomberg predicts that global energy storage will explode from 17GW/34GWh in 2020 to 358GW/1,028GWh by 2030.1
With this expansion on the horizon, the storage sector is rapidly maturing, and we’re seeing more large, late-stage deals to help companies achieve scale. Deals such as Our Next Energy’s $300M Series B demonstrate investors’ interest in the space despite an overall muted venture market.
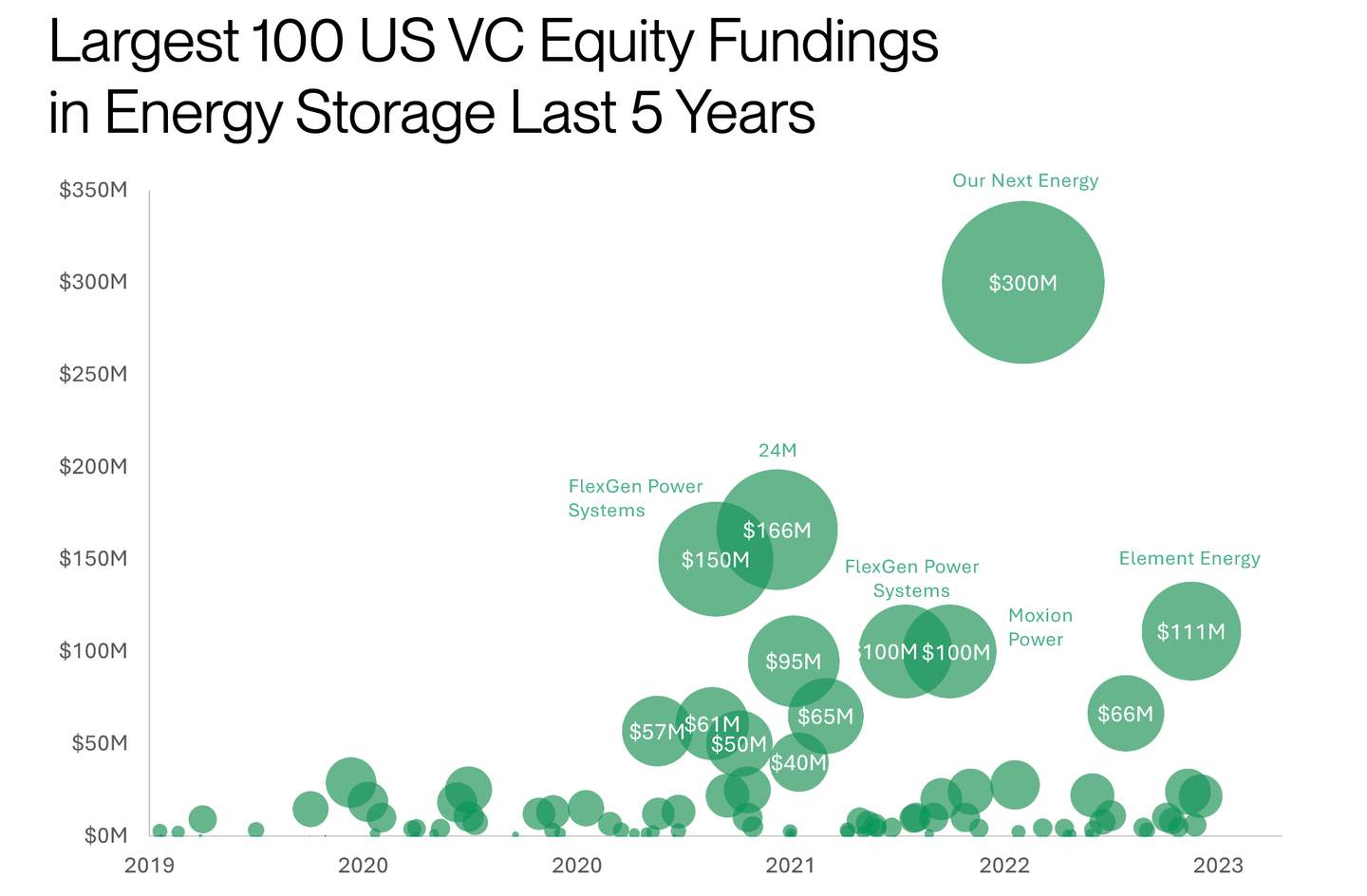
Batteries
While pumped hydroelectric is the most widely used storage technology today, accounting for 94% of grid storage worldwide, batteries comprise the largest share of newly deployed storage systems. Batteries generally have a faster permitting process, the ability to be located where they are needed (not just by water) and are increasingly becoming cost competitive.2
Lithium ion batteries
Lithium Ion (Li-ion) batteries to date have accounted for 95% of grid-scale battery storage systems given their high energy density, easy configuration, voltage range and power ratings. But lithium iron phosphate batteries (LFP) are another configuration gaining market share thanks to their long lifespans, increased safety and low cost. As such, these batteries – at least in the near term – have seen increased penetration for EVs and grid-scale storage. However, neither Li-ion nor LFP batteries are capable of long-duration storage, so other technologies are evolving.
Redox-flor batteries
Redox-flow batteries, developed by companies such as ESS Inc., have gained traction due to their long-term storage capability. The design, consisting of two chemical components dissolved in liquid separated by a membrane, allows for the power rating and the energy capacity to be separately configured. By increasing the size of the tanks, it’s possible to store hundreds of megawatt hours of energy. Though redox flow batteries have high initial costs, these costs are defrayed over the batteries’ extremely long life. Theoretically, a redox-flow battery has an unlimited life cycle with most batteries lasting for years and thousands of cycles. Together, the ability to store a large amount of electricity and a long lifecycle make redox-flow batteries ideal for long-duration storage.
Thermal energy storage systems
Thermal energy storage systems (TESS) utilize heating and cooling processes to store and discharge energy. Depending on the medium and technology, thermal energy can be stored anywhere from hours to months. In some cases it is more energy dense than Li-ion batteries given the high delta in temperature that materials like carbon can achieve. Antora Energy, Malta and Rondo Energy are three notable companies making advancements in thermal energy.
TESS have many practical applications but are particularly well suited to scenarios where energy is needed for thermal end uses, such as residential and industrial heating systems or industrial process heat. According to the Office of Energy Efficiency and Renewable Energy, buildings account for 39% of primary energy and 74% of all electricity consumption in the United States. Approximately half of buildings’ energy demand goes towards thermal end uses (air-conditioning, water heating, etc.).3 One myth associated with TESS is that electricity can't produce high heat. This is not true. Electricity is more than capable of producing sufficient heat; the issue is that it is too expensive.
Thermal storage will enable companies to take excess power from the grid when prices go near zero (or below), store the electricity as heat, and then use it to power industrial processes. This will reduce the need for curtailment and shift demand.
New alternative energy generation sources
Advanced geothermal
Geothermal is by no means new but advances in drilling technologies pioneered by the oil and gas industry have opened a new era of advanced geothermal production. These advances, coupled with government programs such as the DOE’s EarthShots program, aim to reduce enhanced geothermal systems (EGS) costs by 90% by 2035, and have the potential to expand geothermal generation capacity dramatically. If achieved, the advances would bring the cost of EGS down to just $45/MWh according to Bloomberg NEF, making it a critical component of baseload supply. It’s especially true given the high-capacity factors of EGS plants – upwards of 90% – which are only paralleled by nuclear fission. This could make EGS nearly twice as reliable as coal which has a capacity factor of just under 49%.
There are a few core technologies behind advanced geothermal and several different approaches. On the most advanced and highest risk end of the spectrum, you have supercritical geothermal operating in a closed loop. These systems tap into dry tock over 5km below the surface to achieve temperatures greater than 350 degrees F. These projects could be used for electricity generation but also industrial applications, furthering their appeal. Plus, operating in a closed-loop system can enhance the project’s lifespan.
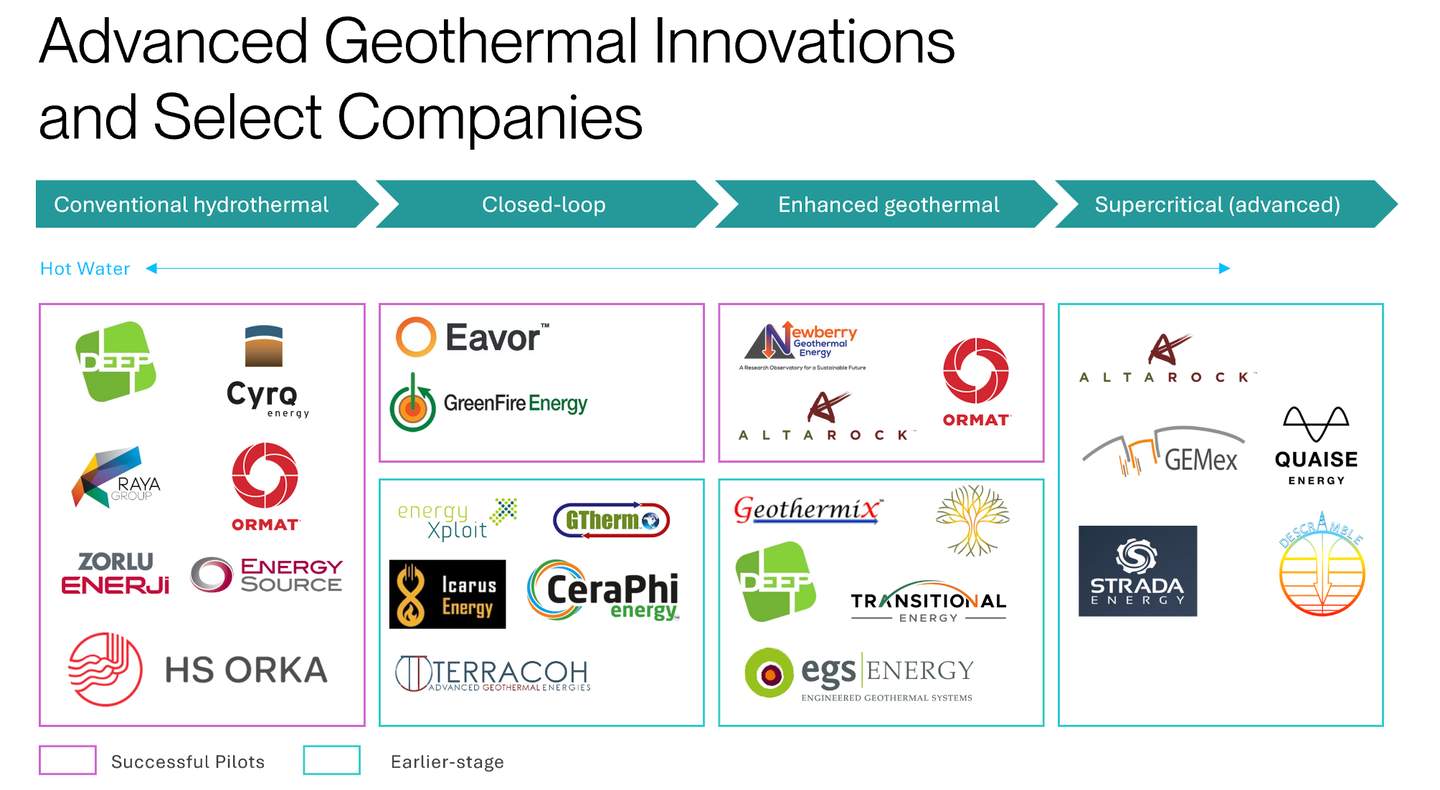
The high capital expenditure required to drill is a barrier for many projects because the risk to the project is still high, making it a tough bet to make for many financiers. For traditional geothermal, drilling typically accounts for about 50% of the overall project cost. For advanced geothermal such as enhanced geothermal systems the cost may increase to 75% as the drilling depths increase.
Despite the challenges to scaling and financing advanced geothermal, we believe the potential for low-cost, reliable power that EGS provides will propel the technology forward.
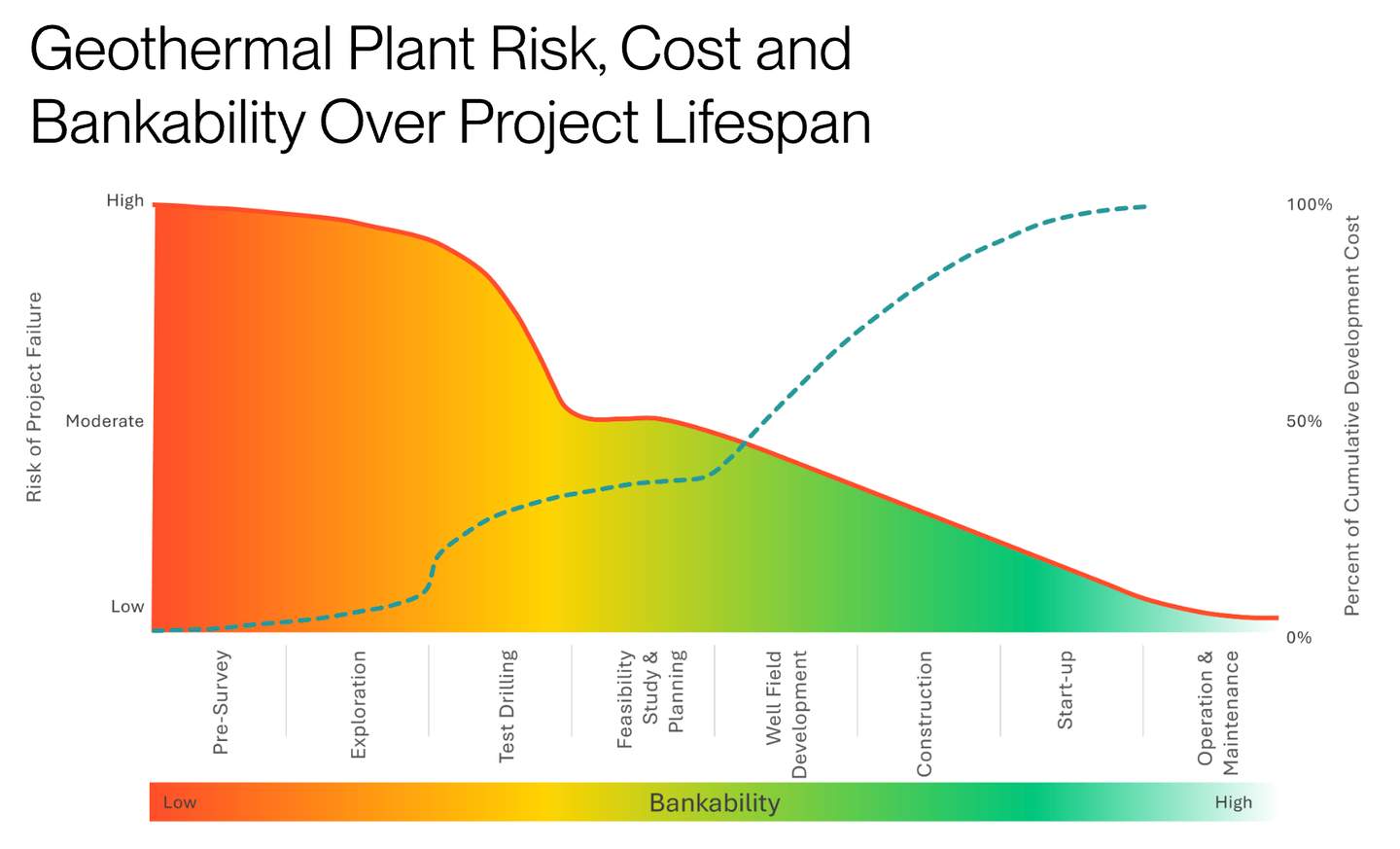
Fission
Because of the events that occurred Three Mile Island and Chernobyl, it’s important to dedicate some time to the topic of advances made in nuclear innovations to address safety concerns regarding nuclear energy production. The “new” or “advanced” nuclear innovations address safety concerns regarding nuclear energy production while being more efficient to build and cost-effective. Entrepreneurs are focusing on smaller form factors using novel coolants, fuels and passive (automatic) safety features to drive innovation in the space.
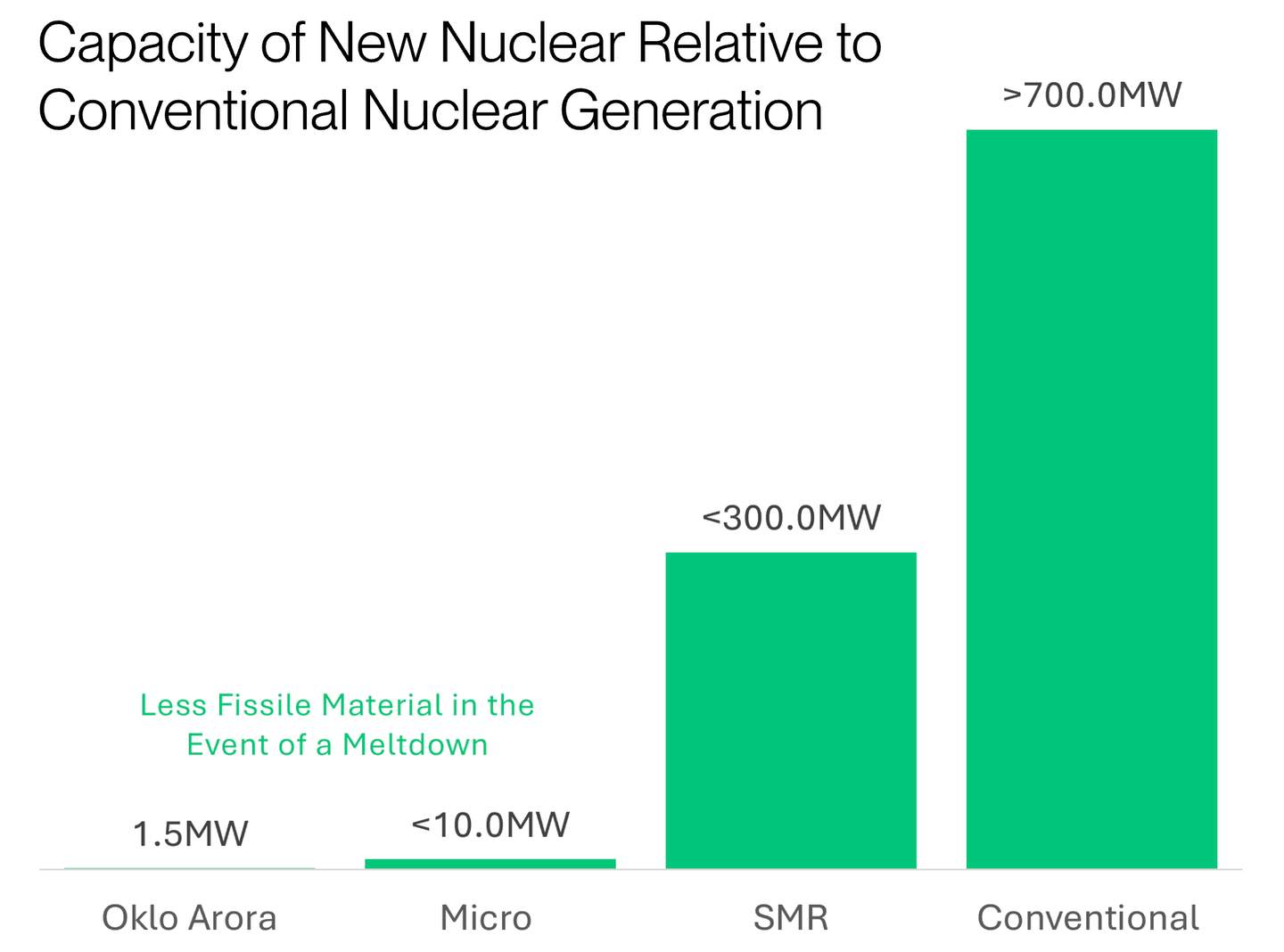
Small modular and microreactors
Light water reactors are the most common nuclear design in the United States, using water as a coolant for nuclear fuel rods. New designs include small modular reactors (SMRs) which are light water reactors 300MW or less in size and micro-reactors which are 50MW or less. While many light water SMRs are under development, only one design by Seattle-based Nuscale Power has been approved to date by the US Nuclear Regulatory Committee. Currently, no microreactor has been approved, yet companies like Oklo are developing reactors as small as 1.5MW.
Molten salt reactors
Molten salt reactors (MSRs) use molten fluoride salts as the primary coolant. They do not produce dangerous and radioactive fission gases that are under pressure, as the gases naturally absorb into the molten salt. Thus, MSRs will likely reduce the risks of radiation leaks making them safer. Molten salts play a key role in the reactor core acting as a coolant instead of using water. Terrapower, Moltex and Terrestrial Power are among the companies currently working on MSRs.
High-temperature gas-cooled reactors
High-temperature gas-cooled reactors (HTGRs) use helium as a coolant. Such reactors can achieve very high fuel utilization rates and operate at high temperatures. This reduces the amount of spent fuel and increases efficiency, thus mitigating some of the environmental and political challenges associated with storing spent fuel. HTGRs also create process heat with myriad applications from hydrogen to chemicals, paper, and food and beverage production. Companies in this space include USNC and XEnergy.
Ultimately, advanced nuclear technologies have the potential to improve safety and reduce costs. SMRs and microreactors can be deployed faster and at a lower cost due to their smaller modular designs that are easier to build and likely easier to permit.
Fusion
Nuclear fusion has fascinated nuclear physicists for decades but only recently (December 2022) did the Lawrence Livermore National Lab achieve net energy gain for a fusion reaction. Fusion is perhaps the technology closest from commercial scale we’ve discussed, but the potential for limitless clean energy is nonetheless captivating. Investors such as Breakthrough Energy Ventures with long time horizons and large sums of capital see the promise in the space, placing large bets on several, well-capitalized companies positioned to find a fusion solution. Notable companies include Helion, Zap Energy, TAE Technologies, Commonwealth Fusion Systems, Renaissance Fusion, Marvel Fusion and Avalanche – all of which have plans to complete demonstrations by 2027. However, timelines for pilots are much further out – 2030 and beyond.
Thus, while the technology holds significant promise, we won’t experience a clean energy panacea overnight. In the meantime, we look forward to seeing the advances in fusion and working with the companies and founders on the bleeding edge of energy innovation.
Marine
While the current generation from marine power is small, only about 500 MW globally, the potential is huge – nearly double the current global electricity demand according to the International Renewable Energy Agency (IRENA). Ocean thermal energy conversion (OTEC) and wave power account for 96% of total potential production, but less than 1% of current deployed capacity, which is tidal power. However, VC-backed companies are recognizing the potential in wave power with 67% of marine power companies developing wave power solutions. Yet many of the top-funded private companies are not based in the US, such as Orbital Marine Power (UK, Tidal power), CorePower Ocean (Sweden, wave power) and Sweetch Energy (France, osmotic power).
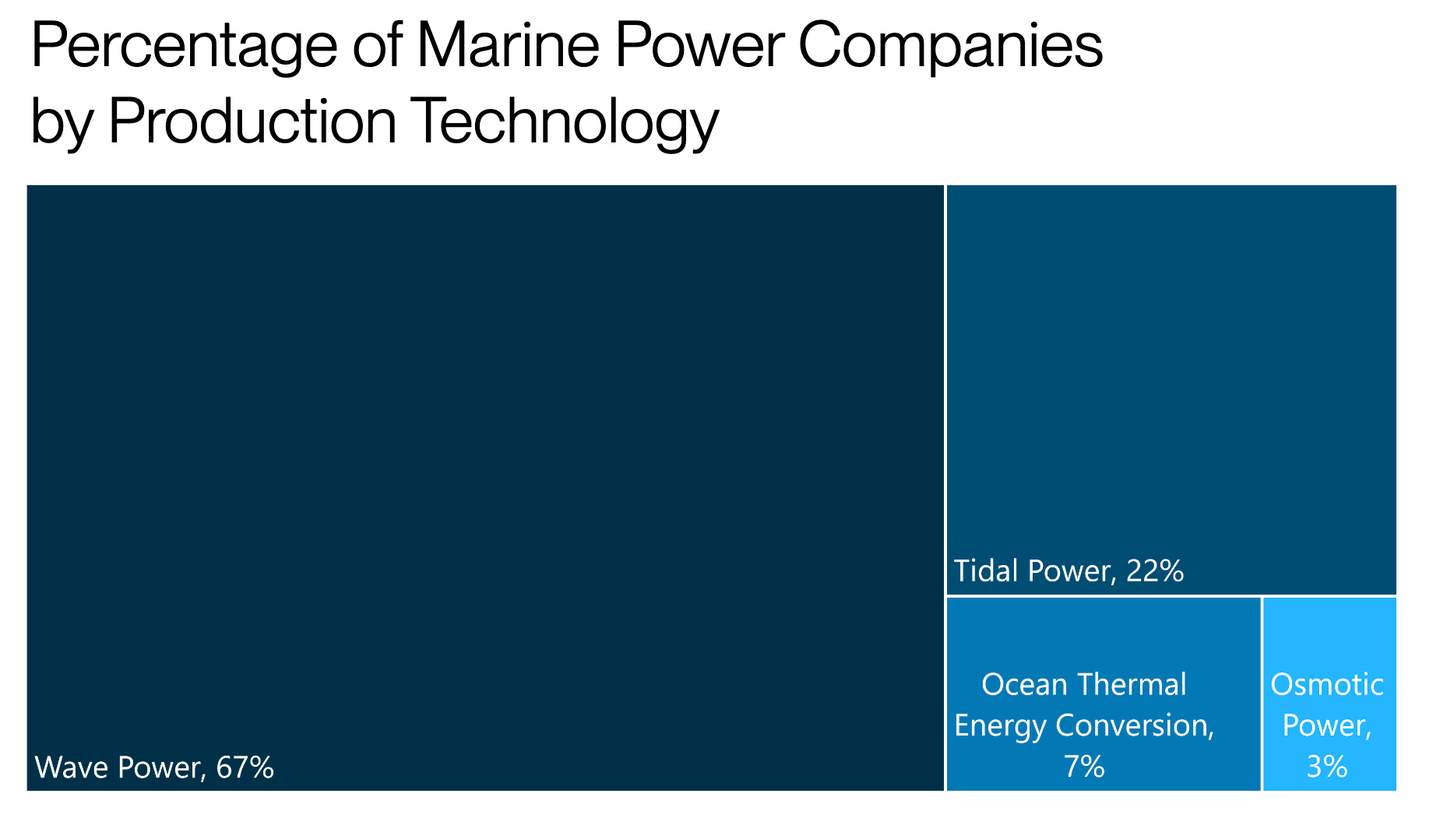
The cost of wave power is high relative to other renewable generation and its technology readiness level (TRL) is lower than tidal power at this point. Given these challenges, many private investors are unwilling to accept the risks without external support such as feed-in-tariffs that would help the technologies achieve economies of scale. Further, hurdles such as a difficult interconnection process to the grid make it uniquely challenging for small companies to build projects.
As a result of these dynamics, overall investment into the space is relatively low. But as technologies scale, the price of wave and tidal power is expected to fall to $118/MWh, according to the EU Strategic Energy Technology Plan, making it cost-competitive with coal ($117/MWh according to their forecasts). Thus, we anticipate increasing penetrations of wave and tidal power which could both provide highly dependable sources to replace current fossil fuel generation.
Final outlook
There is no one silver bullet for efficiently supplying future baseload power. Instead, the solution lies in a diversified grid with enhanced infrastructure using technologies that can balance supply and demand, enhance flexibility and allow higher penetrations of renewable generation while improving system reliability.
Our energy system will face increased stress in the coming decades with more frequent and severe extreme weather events as climate change intensifies. It will be critical for system operators and utilities to implement the latest technologies while at the same time achieving climate goals and reducing carbon emissions.
Sitting at the nexus of finance, climate technology and energy, we at SVB will continue to serve as a financial partner to many of the world’s most promising climate tech companies. We look forward to collaborating with innovators in this space and watching the technologies of tomorrow mature.